Circular Economy and Me – Issue 11

How Can We Reduce Plastic Waste? By Dr Donald R Inns
Man-made plastics have become a ubiquitous part of our daily lives and are found in almost every product, owing to their tailorable, mouldable, and durable properties. The majority of these plastics are made from monomers derived from fossil fuels, such as ethylene, propylene, and styrene. Thus, plastics not only contribute to fossil fuel depletion but are not biodegradable. Therefore, plastics accumulate rather than decompose and whilst they remain integral to many applications it is now clear that single use plastics, such as those from packaging, coupled with the large-scale production of plastics is leading to unmanageable amounts of waste. An estimation of global plastic production, use, and its fate from 1950 to 2015 in million metric tons (Figure 1) highlights the scale of the issue and the vast amount of plastic waste that has been discarded [1].
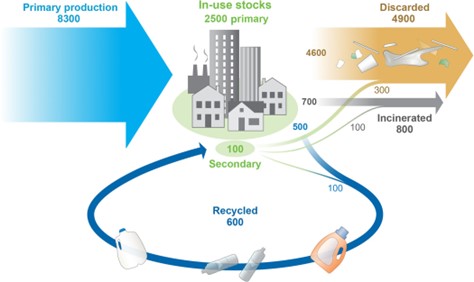
Given this context, an important question arises, namely: “How can we reduce plastic waste?” and whilst this may seem a simple question, it requires a complex set of answers that are multi-disciplinary, requiring policy, social, scientific, and engineering aspects to coalesce for circularity of the plastic economy. This is particularly important as the answers require not only to address current plastic production and new waste, but also existing waste, which is causing great environmental damage and pollution.
The social and policy aspects can be used to influence the type of plastics used and the amount of waste that is produced through changing attitudes and laws to regulate plastics. However, the current main methods of reducing plastic waste (physical recycling and incineration) cannot be used to completely eliminate the production of plastic waste. Especially as incineration leads to the release of CO2 into the atmosphere and provides limited energy gain. Physical recycling is and will increasingly become a key part in the circularity of plastics, but it is energy and time intensive and some plastics such as polypropylene have extremely low recycle rates. Therefore, new innovations are needed to improve the circularity of the plastic economy. Such innovations include new plastics from sustainable precursors, biodegradable plastics, and new methods for the recycling of plastics.
As part of the National Centre for the Circular Chemical Economy, our team within the Rosseinsky group at the University of Liverpool, in conjunction with computational teams at Cardiff University, is developing new catalyst materials for the chemical recycling of plastics to break down polypropylene into liquid alkanes via hydrogenolysis [2]. The hydrogenolysis process (Figure 2) utilises catalysts and hydrogen to cleave backbone C-C bonds and generate mixtures of short chain alkanes in the form of an oil or wax. Polypropylene was chosen as a particularly problematic plastic that has low global recycle rates and is used extensively in packaging and domestic products. As such, we applied this process to both pristine and consumer polypropylene such as file sheets, centrifuge tubes and fragrance cases. Additionally, green hydrogen can be used in hydrogenolysis and can be generated from circular processes such as electrolysis or from biomass [3].

Our catalyst design strategy is based on nano-shaped materials (nanocube or nanorod CeO2) to support clusters of ruthenium atoms. The small clusters of ruthenium are highly active in activating hydrogen and cleaving internal C-C bonds, which in-turn, increases the rate of formation and yield of liquids. Whereas it was found that larger particles of Ru on the non-shaped catalyst cleave C-C bonds at a lower rate and cleave C-C bonds at the ends of chains (terminal) to generate the gas product methane. Additionally, the shaped materials increase the availability of hydrogen for cleavage by increased spillover of hydrogen onto the support upon its activation on the ruthenium. This leads to the shaped catalysts achieving vastly improved depolymerisation activity using a lower amount of expensive active metal.
Another important aspect of the research found that the liquid product chain lengths could be tuned using the reaction conditions (temperature, metal loading, pressure, and time). This means we can tune the physical properties, such as viscosity, of the oils. This strategy is envisaged as being key to widening the applications of the liquid products to include re-polymerisation, chemicals, oils, and lubricants. Therefore, the hydrogenolysis chemical recycling process means we can reuse the plastics in another form, utilising the locked carbon in waste products whilst decreasing the net carbon contribution of other supply chains and removing dangerous solid waste from the environment. Thus, improving the circularity of the plastic economy and potentially other economies.
References: